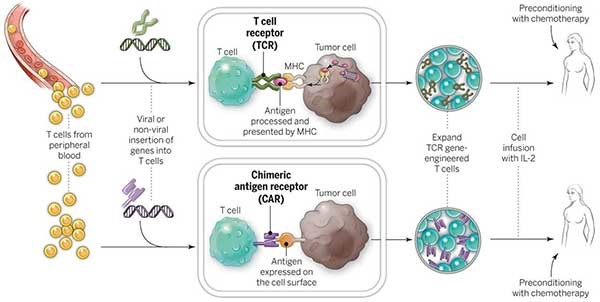
Fighting acute lymphoblastic leukemia (ALL) with CAR-T regenerative medicine
Chimeric antigen receptor T-cell (CAR-T) therapy is a rising shining star in Regenerative Medicine. Medical devices will be involved in many of the processes involved with CAR-T therapies. From blood collection, to T-cell isolation and activation, modification, expansion and product formulation, preconditioning and finally T-Cell infusion and delivery, there will be an evolution in the associated medical devices to support a treatment worthy of a ‘miracle cure moniker’.
Acute Lymphoblastic Leukemia is a form of cancer that originates in the blood within a subtype of white blood cell called a lymphoblast. It is the most common type of childhood cancer, with a high relapse rate of 15-20%. Relapse patients are typically unresponsive to conventional therapies such as chemotherapy, radiation and surgery, prompting the need for an alternative approach.
A new therapy termed chimeric antigen receptor T-cell (CAR-T) therapy uses a one-time infusion of the patient’s own genetically modified white blood cells to destroy cancer cells, with an 83% remission rate after 3 months and a 62% relapse-free survival rate after 24 months. CAR-T cell therapy treatment [KYMRIAH® (tisagenlecleucel)] was developed by Novartis at the Hospital for Sick Kids in Toronto for use on patients up to 25 years of age. It was approved by Health Canada in September 2018, and by the U.S. Food and Drug Administration (FDA) in 2017. The therapy is unique in its ability to overcome the mechanisms used by cancer cells to avoid recognition by the T cells of the immune system.
The adaptive immune response is primarily composed of two white blood cell subtypes – B cells produced in bone marrow, and T cells produced in the thymus. While B cells respond to foreign particles (antigens) by producing antibodies to mark the cell for destruction, T cells respond directly by inducing apoptosis on the cell – programmed cellular death. Unlike B cells, T cells are unable to directly recognize antigens and must rely upon helper cells, termed Antigen-Presenting Cells, to present antigens by way of protein complexes called major histocompatibility complexes (MHCs).
T cells bind to MHCs and the presence of an antigen is relayed to the T cell through a second protein complex called CD3, leading to activation of the T cell. Activation by MHC binding is enough to arm T cells, but insufficient to promote their expansion and survival. This generally leads to a weak immune response. A strong response requires additional signaling by co-stimulatory molecules. Thus once a T cell has bound to both an MHC and co-stimulatory molecule, it becomes activated towards a specific antigen and expands its numbers to effectively neutralize the threat.1
Cancer cells avoid T cell recognition through several mechanisms including downregulating MHC and co-stimulatory molecule expression.2 CAR-T cells are T cells sourced from a patient’s blood that are genetically modified to target lymphoma cells, bypassing the need for activation through MHCs and co-stimulatory molecules. Using gene transfer technology, genes are inserted into the T cells encoding CD19 – an antigen specific to lymphoma and lymphocytic leukemia, CD3 – the signal transduction complex that activates the T cell, and CD137 – a co-stimulatory molecule. This combination effectively activates the T cells to destroy CD19-expressing cells, while the CD137 co-stimulatory signaling ensures the expansion and survival of the modified T cells upon re-infusion into the patient.3-4
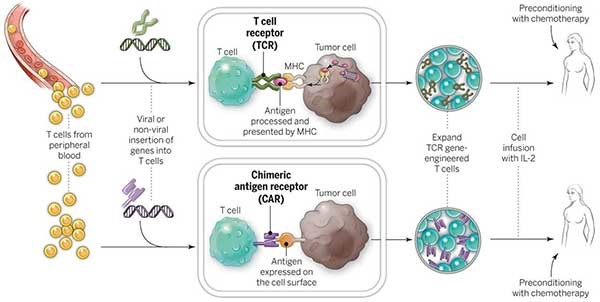
Although promising, there are potential side effects of CAR-T cell therapy that need to be managed. The most common side effect is cytokine release syndrome (CRS), which occurs when cancer cells die in large numbers, releasing their contents into the blood stream. CRS can be mild or life threatening. It is treatable with Actemra (tocilizumab), an IL-6 receptor antagonist, with a success rate of 69%. Other serious side effects include neurological events, and “in target/off-tumor” recognition (hypogammaglobulinemia), wherein healthy CD19-expressing B cells are destroyed leading to an increased susceptibility to infection. These toxicities occur typically between days 1-14 and become very rare after 8 weeks. Despite these limitations, CAR-T cell therapy is a promising and quickly growing field of immunology research, with new approaches to manage toxicity and design better CAR-T cells emerging every day.
While scientists figure out how to overcome these technical challenges, and regulators struggle to define how CAR-T cells fit within several regulatory frameworks, medical device engineers will continue to work to support CAR-T therapies along the entire pathway. From blood collection, to T-cell isolation and activation, modification, expansion and product formulation, preconditioning and finally T-Cell infusion and the delivery of the miracle cure known as CAR-T, medical devices will be part of the solution.
- Driessens, G., Kline, J., & Gajewski, T. F. (2009). Costimulatory and coinhibitory receptors in anti‐tumor immunity. Immunological reviews, 229(1), 126-144.
- Sharma, P., Hu-Lieskovan, S., Wargo, J. A., & Ribas, A. (2017). Primary, adaptive, and acquired resistance to cancer immunotherapy. Cell, 168(4), 707-723.
- Porter, D. L., Levine, B. L., Kalos, M., Bagg, A., & June, C. H. (2011). Chimeric antigen receptor–modified T cells in chronic lymphoid leukemia. New England Journal of Medicine, 365(8), 725-733.
- Milone, M. C., Fish, J. D., Carpenito, C., Carroll, R. G., Binder, G. K., Teachey, D., … & Campana, D. (2009). Chimeric receptors containing CD137 signal transduction domains mediate enhanced survival of T cells and increased antileukemic efficacy in vivo. Molecular therapy, 17(8), 1453-1464.
Meghan Robinson is a part-time StarFish Medical BioMedical Engineering Lab Technician. In addition to her work with StarFish Medical, Meghan researches cell reprogramming under Dr. Stephanie Willerth at the University of Victoria and is the lead author on a number of peer reviewed publications in this field.
Images: StarFish Medical