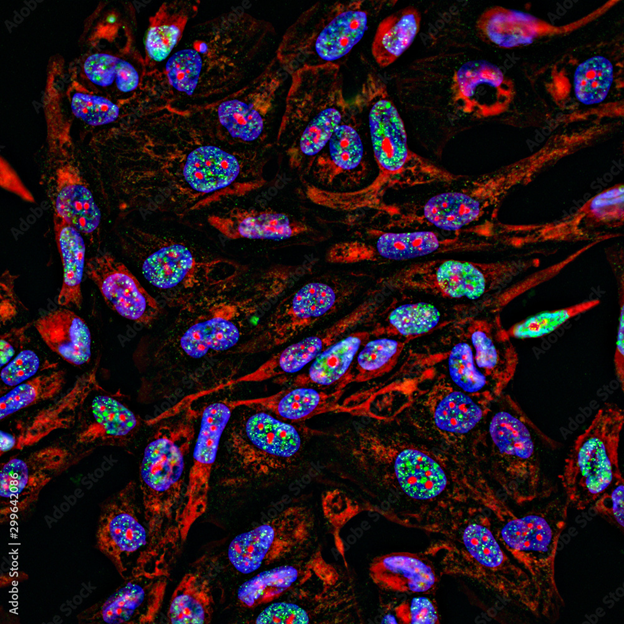
Fluorescence Imaging in Medical Devices – Applications and Examples
Fluorescence Imaging in Medical Devices
Fluorescence from cells or tissues is commonly used to create images and video in a clinical setting. Ensuring appropriate imaging characteristics, including those that specifically relate to the imaging and/or quantification of fluorescence are key to success in the design of fluorescence-based imaging devices. This blog outlines various medical applications of fluorescence imaging, examples of different types of devices that use fluorescence for imaging, and finally some key performance characteristics that are important in medical fluorescent imagers.
Medical Applications of Fluorescence Imaging
Fluorescence is a process in which a material absorbs light and then subsequently emits light of a different wavelength, making the substance glow with a colour different than the light it absorbed. A variety of fluorescent substances (or “fluorophores”) are used in medicine. Medical techniques that use fluorescence involve incorporating a fluorescent dye (or a precursor) into a biological sample and taking images or video of the sample with the fluoresced light isolated. Such imaging methods are often used to isolate biological structures or substances on which a fluorophore is known to accumulate or attach.
Fluorescence imaging is used in several contexts in medicine. These include surgical guidance, the mapping of fluid flows within the body, ophthalmic examinations, and cell counting and imaging techniques.
Certain fluorescent molecules, such as protoporphyrin IX, can be made to selectively accumulate in cancer cells, which is useful in the surgical excision of malignant tissues. Prior to surgery, a dye (or a precursor) is administered to the patient intravenously, topically, or orally, and subsequently accumulates in tumor tissue. This causes the tissue to fluoresce when illuminated with light of a certain wavelength (i.e. colour). The observed fluorescence can be used to help identify the margins of the tumor, allowing the tumor to be removed precisely and reducing the risk of malignant tissue being left behind.
Fluorescent dyes injected into a patient’s tissue or fluid vessels, such as indocyanine green (ICG), can be used to visualize fluid flows (i.e. perfusion) within the body when illuminated. In oncology, the visualization of lymph flow is used to identify lymph nodes nearest to a tumor site. These are the nodes that cancerous cells are most likely to travel to from the original tumor site, and therefore the best candidates for biopsy. Similarly, fluorescent dye injected into the blood can be used to visualize blood flows. This visualization may be used to ensure adequate blood flow into transplanted tissue, to identify abnormalities in blood vessels, or to identify abnormal functioning of the heart.
In ophthalmological examinations, a fluorescent dye, fluorescein, can be topically administered to the surface of the eye for several types of tests. These include measurement of the time for the eye’s tear film to form dry areas (which can indicate dry eye disease), the identification of corneal abrasions in which the dye tends to accumulate, and for contact lens fitting. Additionally, intravenously injected ICG can be used to identify abnormalities in the blood vessels of the retina, which may indicate macular degeneration or other pathological findings.
Finally, fluorescent tracers can be made to bind to specific cell structures or disease markers in cells. Fluorescence can make certain structures of the cells more visible. Abnormalities in the shape or size of some cells may indicate diseases such as cancer. Fluorescent tracers can also be made bind to certain types of cells or disease markers in or on the surface of cells, such as antibodies. Subsequent analysis of the number of cells exhibiting florescence in a patient sample (by flow cytometry or fluorescence microscopy, for example) may then indicate the presence of immunological, bacterial, or viral pathologies.
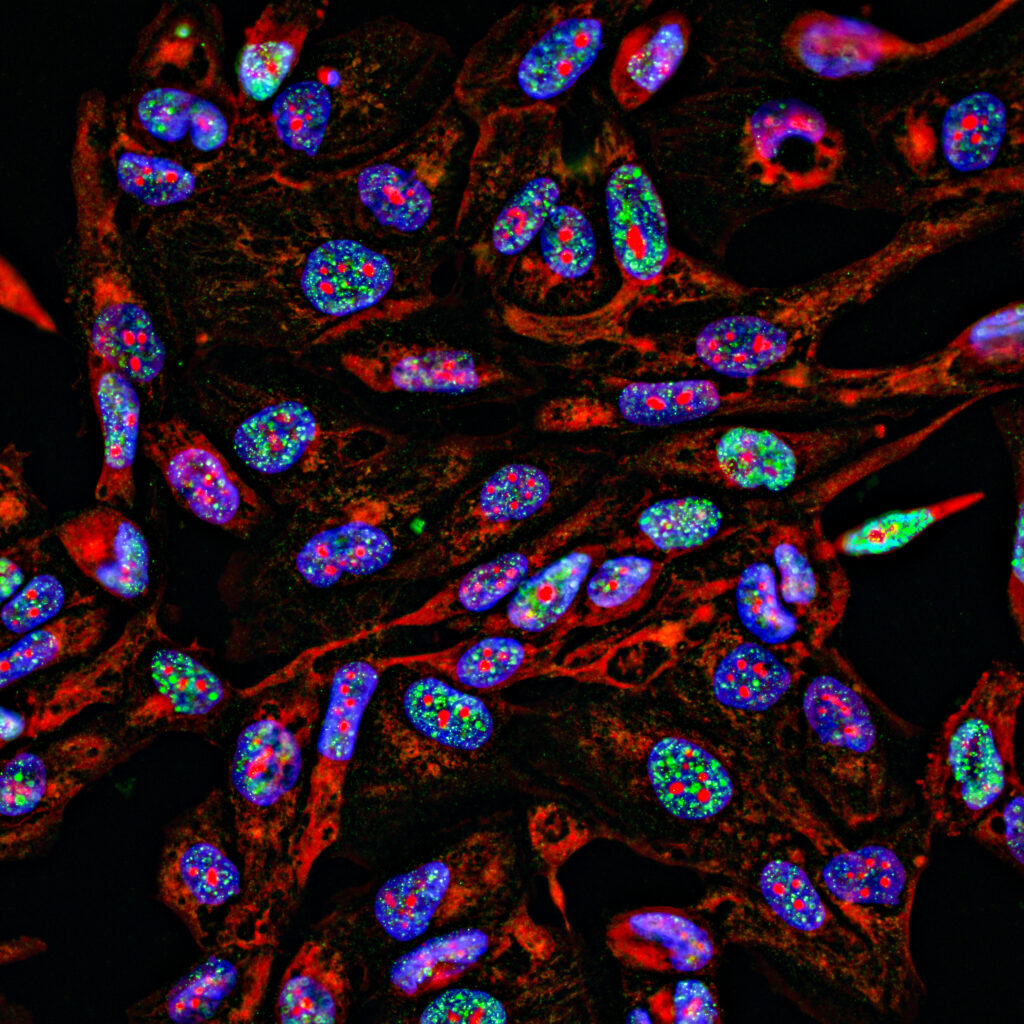
Types of Fluorescence Imagers
Fluorescence-imaging camera systems resemble normal cameras but typically incorporate an illumination system at a wavelength appropriate to excite the target fluorophore, as well as a colour filter on the camera to isolate the fluorescent light for imaging. Often, such imagers are used with dyes that absorb and emit infrared light that penetrates deeper through biological tissues than visible light. As a result, these imagers are sometimes able to take images or video of internal structures of the body containing a fluorescent dye – such as ICG injected into the blood vessels – without needing the camera to be inside the body.
Fluorescence microscope systems operate analogously to visible-light microscopes but use illumination systems that excite fluorescence and imaging systems that isolate the emitted light, similar to fluorescence imaging cameras. Wide-field fluorescence microscopes can be used to image fluorescence from relatively large tissue samples or to analyze many fluorophore-traced cells simultaneously. Medical uses of such microscopes include detection of pathogens using fluorescent tracers, and histopathology of resected tissue samples.
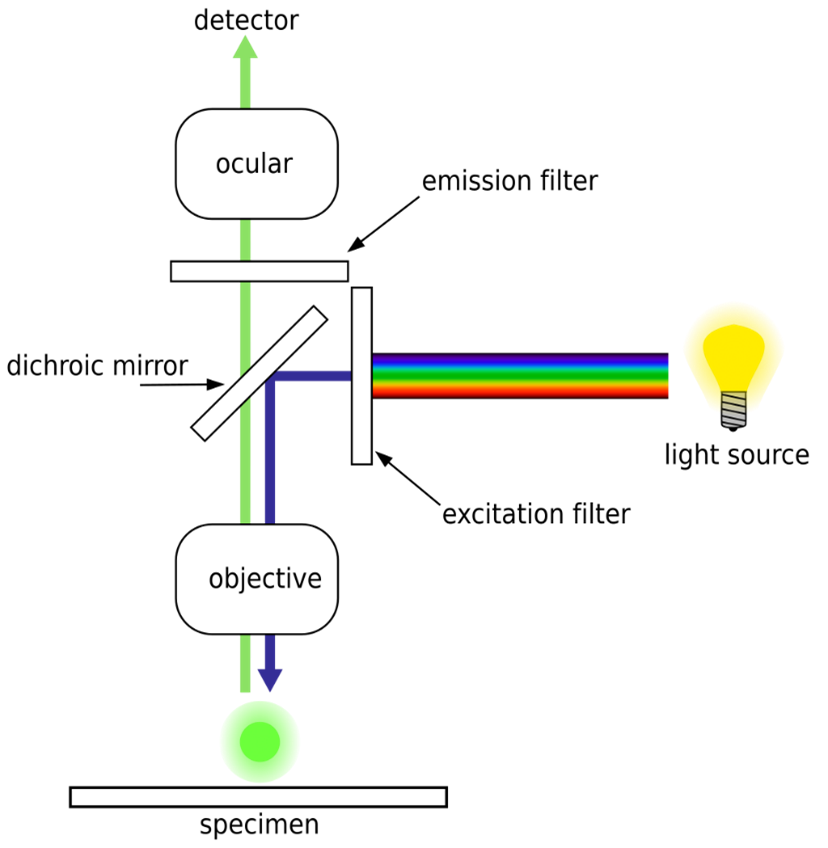
Two types of microscope systems whose fluorescence-based variants are widely used in medical research are confocal laser scanning microscopes (CLSM) and total internal reflection fluorescence microscopes (TIRFM).
Fluorescence-based CLSM systems use a focused point of laser light on a sample to excite fluorescence and use a specialized imaging system to isolate the fluorescence from the location and depth that the laser was focused. The laser spot is then scanned over the target to reconstruct a 2D image of fluorescence from the sample at a specific depth. 3D reconstructions of samples are also possible by scanning at multiple depths. In addition to applications in medical research, fluorescence-based CLSM can be used for histology of fresh ex-vivo patient samples (such as examining margins of excised fluorescent-labelled tumors).
TIRFM uses total internal reflection in a substrate on which samples are mounted to excite fluorescence in only a very thin (<200 nm) section adjacent to the substrate. TIRFM is often used to image and study processes that occur on or near cell membranes. In medicine, TIRFM is primarily used as a research tool.
Several types of devices are used for fluorescence-labelled cell counting, which can be used to diagnose or monitor diseases such as leukemia or HIV in patient samples. Fluorescence wide-field microscopes, when used to view a large number of fluorescent-tagged cells, are one such example.
Fluorescence-based flow cytometers, while not technically an imaging method, are a common device for counting fluorescent-labelled cells in a patient sample. Such flow cytometers work by shining a laser on individual cells as they pass through a narrow channel. A light detector placed near the channel is filtered such that it only detects light from fluorescence.
Fluorescence is observed when the cell on which the laser is incident contains a certain fluorescent analyte. By applying this method to many cells, the proportion of cells which are fluorescent-labelled in a patient sample can be determined.
Requirements of Fluorescence-Based Imagers
Depending on their intended application, the characteristics of a given fluorescence-based imager will need to meet certain specifications. Some of such requirements are common to all types of imaging systems, while others are specific to fluorescence-based imagers.
Similarly to other camera or microscope systems, the general imaging requirements of a fluorescence-based system must be understood.
The field of view (FOV) of a fluorescence imager, which refers to the size of the field that is imaged, must be sufficiently large for the samples under study. The required FOV may be less than one millimeter, such as when single cells are being studied, or as large as centimeters, such as for the study of large, excised samples.
The imager’s resolution refers to the smallest feature that can be reliably imaged, which must be sufficiently small to view any feature of interest of a sample.
The depth of field (DOF) refers to the depth over which an image appears to be in focus. Some applications, such as imaging of fluorescence in a surgical field may require a DOF of at least several centimeters, while other imagers, such as CLSM devices, may have a very shallow effective DOF to allow for different layers of a semi-transparent sample to be imaged in isolation.
Image distortion refers to deviations in the angles or dimensions of an object when it is imaged, usually due to the imager’s lens system. Such distortion must be limited in cases where the image of an object must maintain spatial accuracy.
Certain imaging characteristics are specific to fluorescence-based imagers, the requirements for which must also be understood. The ability to detect a certain known concentration of a fluorescent substance in a sample is of utmost importance.
The imager’s sensitivity refers to how measured brightness of a fluorescent sample scales with the concentration of a fluorophore in a sample. The sensitivity must be such that the relevant range of fluorophore concentrations can be detected and sometimes quantified.
The imager’s limit of detection (LOD) refers to the smallest concentration of a fluorophore that can be distinguished from zero, and limit of quantification (LOQ) which refers to the smallest concentration that can be quantified.
The imaging system’s linearity refers to how the detected brightness correlates with a certain concentration of fluorophore. If a fluorescence-imaging system is linear, the brightness will vary directly in proportion with the concentration of the fluorophore (for example, twice the concentration would result in twice the observed brightness). It should be noted that, at sufficiently high concentrations, some fluorophores themselves exhibit non-linearity in the emitted brightness (“fluorescence self-quenching”).
For fluorescence-based imagers that take images at a certain depth in tissue, additional characteristics may apply. The sensitivity may be reduced if the fluorophore is under a layer of tissue. Therefore, it may be important to understand the sensitivity as a function of tissue depth for a given concentration. Furthermore, scattering in tissue of emitted light from a fluorophore at some depth may blur the image of the fluorophore, leading to reduced effective resolution. Therefore, it may also be important to quantify the resolution as a function of tissue depth for the imager.
Conclusion
From surgical guidance to disease diagnosis and monitoring, to histopathology of excised tissue, fluorescence imaging has a wide variety of uses in medicine and medical research. Fluorescence-based cameras, microscopes, and cell counters each have multiple uses that ultimately contribute to better patient outcomes. The design of effective fluorescence-based medical imaging devices requires certain imaging specifications to be met, including those that specifically relate to the imaging and/or quantification of fluorescence.
Ryan Field is a Senior Optical Engineer at StarFish Medical. Ryan holds a PhD in Physics from the University of Toronto. As a post doctoral fellow, he worked on the development of high-power picosecond infrared laser systems for surgical applications as well as a spectrometer from home materials.
Overview of Optical Technologies for Point of Care Medical Devices