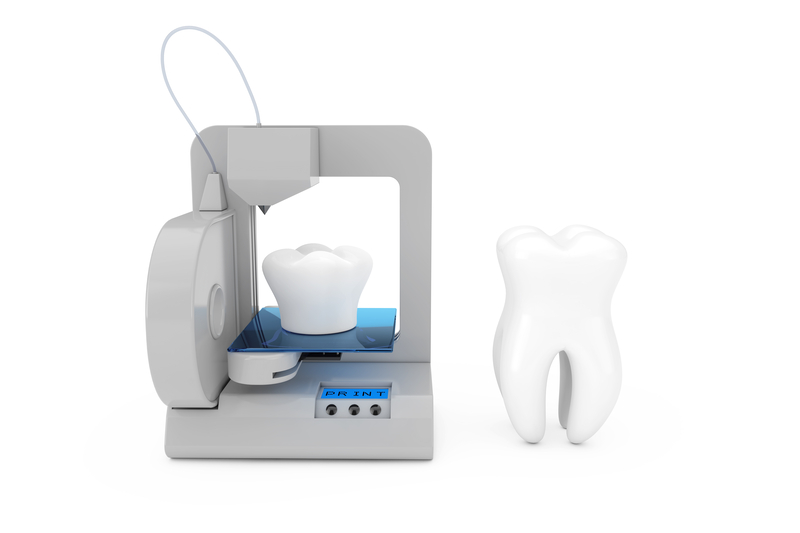
3D Printed Parts Streamline Development Process
This blog provides a context for designers to streamline their development process using 3D printed parts as a part of their market-ready medical devices. 3D printing is disruptive. It challenges the way that designers consider product development. Contrasted with traditional fabrication methods, 3D printing offers several benefits along with certain limitations. Regulatory agencies are providing guidance to 3D printed component testing and process control, which allows for 3D printed parts to be included in medical devices once they are defined by the manufacturer.
Pros and Cons that help determine when to switch to CAD
3D Printing vs. Traditional Fabrication Comparison
Compared to subtractive and injection molding (IM), 3D printing offers:
- Multitude of material choices and mechanical properties (elastomeric, thermoplastic, thermoset, composites, in-fill [glass, carbon fiber])
- New options with internal and external geometries
- Material density control
- Opportunity for faster design iterations/test cycles
- More cost effective at lower volumes
For example, a 3D print with the multi-step setup for machining plastic parts is cheaper and faster than the costs and timeline of an IM part mold fabrication.
There are a few process limitations, including:
- Limits to quantity per print, due to:
- Bed size limit
- Material height limit
- Material-specific storage issues, such as:
- Light sensitivity (crazing, discolouration)
- Degradation in ambient air
- Humidity intolerance (swelling, bubbling and printer head issues)
- More parameters to configure during a build, including:
- Ambient print temperature
- Humidity
- Print bed area variation in accuracy (centre to edge)
If printing parameters slip from their specified tolerance, they could lead to issues in part quality, including mechanical property changes and unintended material deformation.
Please see my previous 3D printing blog for a comparison of 3D printing fabrication methods, pros/cons, and applications.
Product Inclusion
3D printing is frequently used in early device development, from early form discovery and formative studies to technology de-risking through early stage testing. However, being able to carry a tested 3D printed part through to a production-level component is alluring. There are several considerations to this.
- Volume. If this is known at an early stage, it will chart a path to a given process for the part in question. If the part or component is critical enough to merit it, a COGS analysis comparing 3D printing to other processes should be performed – my colleague Nigel Syrotuck breaks this down thoroughly in his blog.
- Part complexity and variation. One alluring aspect of 3D printing is the ability for each print to be of a different shape. For medical devices, unique patient anatomies may require a per-patient design. 3D scans of the patient can be leveraged to form 3D printed parts to suit this need. One example of this is with Invisalign’s clear overlaid braces. Each set of braces is used for a period of around two weeks, then discarded and replaced with the next set using a different form guiding teeth to their new position. 3D printing not only allows for form variation but provides cost targets for the braces that enables each treatment to be individualized to a patient treatment plan. Invisalign and other clear braces treatments (their patents have now expired) have eclipsed traditional orthodontics for many patients. They no longer have to contend with wire braces, retainers, and head gear, all thanks to 3D printing technology.
- Part requirements. Medical device development relies entirely on the design history file executed and described via the requirements, specifications and architecture. As an output, verification plans define the test required to prove the device’s suitability for use in it’s final application. Some example tests could include:
- Bringing the part to yield and ultimate strength against a required safety factor
- Exposing the part to specified cleaning fluids, determining the material compatibility
- Wiping the device with gloves or specified cleaning wipes, determining acceptable sharps and corners
- Testing for specified sealing rating, through and between parts
Once a device passes all required tests and the device is approved through applicable regulatory bodies, such as the FDA and Health Canada, it can begin transfer to manufacturing.
For full details on the FDA’s guidance for 3D printing, please see my previously published blog, which includes a link to the published guidance.
3D Printed Parts in DFMA and Manufacturing
Using principals of Design for Manufacture and Assembly (DFMA), engineers can include updates to component and assembly design for improved scale and assembly. The human factors aspect of assembly increases as the volume of assemblies grows and from learnings during prototyping can be applied.
Examples where 3D printing can help in manufacturing environments include:
- Merging parts to reduce:
- Part count
- Assembly error
- Labour
- Failure points
- Lower material waste
- Environmental benefit (energy savings, recycling options)
- Increased cleanliness (no lubricant and coolant)
- Integrated fastening strategies (e.g. clips, heat stakes)
Lower constraints on the geometry of 3D printed components opens opportunities to integrate several parts into one and lower the part count. The ability to optimize material density through internal lattice or honeycomb structure allows for parts that appear solid but weigh less.
Overall, NPI improvements seek to lower Non-Compliance on incoming inspection of components and ongoing assembly. NPI redesign work leads to a smoother transition into the manufacturing department. Leveraging 3D printing, NPI engineers can maintain system function and safety while creating a design that allows for more consistent quality in volume. 3D printing can also lead to increased efficiency during assembly, providing cost savings in volume and over time.
Conclusion
While 3D printing has provided new opportunities and challenges for designers in product development, it can also fit neatly into an established workflow of bringing products to market. New regulatory guidances on 3D printing process controls for medical devices are encouraging the industry to explore what 3D printing can apply to real-life products. Significant design opportunities create a chance to lower costs and increase device quality throughout the product’s development pathway.
Nathan Müller, EIT, is a StarFish Medical Mechanical Engineer. He is passionate about the applications of biotechnology and biomedical science in product development, with core competencies in biomedical-mechanical design and material sciences.
Illustration 140389681 © Alexander Kharchenko | Dreamstime.com
Learn how patient imaging data can be a powerful and robust source for developing anatomically accurate and functional 3D models.
How to get the most from your FDM 3D printer.